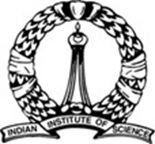 |
Optimal Treatment of Domestic Wastewater through
Constructed Wetlands
|
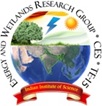 |
Results and Discussion
Lake water is being used for domestic purposes, irrigation, recreation, etc. Lake is also the source of livelihood as it support fishing and washing activities. Monitoring of water quality would help in maintaining the quality and sustainable management of the ecosystem. Water sampling was done at stations and parameter wise results for sampled locations are listed in Tables 2 (onsite parameters), Table 3 (chemical parameters) and Table 4 (nutrient parameters) respectively and each parameter variability at sampling locations have been discussed next.
Dissolved Oxygen
Dissolved oxygen (DO) is the most essential feature in aquatic system that helps in aquatic respiration as well as detoxification of complex organic and inorganic mater through oxidation. The presence of organic wastes demands high oxygen in the water leading to oxygen depletion with severe impacts on the water ecosystem. The DO of the analyzed water samples varied between 0 to 17.74mg/l. The higher variations of DO especially lower DO values are indicative of high organic matter in the immediate vicinity. The DO was very low at the inlets of wetland and increased immediately after the algal pond. Lower DO values were observed near the macrophytes (invasive exotic weeds as water hyacinth, Eichhornia crassipes) infested regions at the outfalls outlets.
Total Dissolved Solids (TDS)
Total dissolved solids present as mineral matter in the form of dissolved cations and anions and to a smaller extent by organics, sourced from decomposing matter. Other sources include runoff from urban areas, road salts used on street, fertilizers and pesticides used on lawns and farms (APHA 2017). TDS affect the water quality in many ways impacting the domestic water usage for cleaning, bathing etc. as well as drinking purposes (Ramachandra et al. 2012b). Surface as well as groundwater with high dissolved solids are of inferior flavor and induce an unfavorable physiological reaction to the dependent population. The TDS values in the samples analyzed, ranged from 612 to 710 mg/l across all locations. It was higher in the inlets and reduced in the middle region of the lake. The TDS was little higher in the outlets than middle due to human activities (like washing, etc.), macrophyte and plankton cover etc.
Table:2 Onsite Parameters
Table:3 Chemical Parameters of Water Analysis
Table:4 Nutrient Analysis of Water
pH
pH is a numerical expression that indicates the degree to which water is acidic or alkaline, with the lower pH value tends to make water corrosive and higher pH has negative impact on skin and eyes. The pH value ranged from 7.2 to 8.4.
Chlorides
Chlorides are essentially anionic radical that imparts chlorosity to the water. An excess of chlorides leads to the formation of potentially carcinogenic and chloro-organic compounds like chloroform, etc. Chloride values in samples collected from Jakkur lake system ranged from 166-260 mg/l. Chloride values were high at inlets (treated and untreated water) and relatively lower at the outlet of algal pond and the middle portion of Jakkur lake. At outlets, it is higher due to washing activities with the use of bleaching powder that is CaO (Cl)2.
Sodium
Sodium (Na) is one of the essential cations that stimulate various physiological processes and functioning of nervous system, excretory system and membrane transport in animals and humans. Increase of sodium ions has a negative impact on blood circulation, nervous coordination, hence affecting the hygiene and health of the nearby localities. In this study, the concentration of sodium ranged from 256 to 367 mg/l and higher values were observed in samples collected at outlets.
Potassium
Potassium (K) is an essential element for both plant and animal nutrition, and occurs in ground waters as a result of mineral dissolution, decomposing of plant materials and also from agricultural runoff. Potassium ions in the plant root systems helps in the cation exchange capacity to transfer essential cations like Ca and Mg from the soil systems into the vascular systems in the plants in replacement with the potassium ions (APHA 2017). Incidence of higher potassium levels in soil system affects the solute transfer (active and passive) through the vascular conducting elements to the different parts of the plants. The potassium content in the water samples ranges between 47-58 mg/l. The potassium values were high at outlets due to decomposition of plant materials.
Alkalinity
Alkalinity is a measure of the buffering capacity of water contributed by the dynamic equilibrium between carbonic acid, bicarbonates and carbonates in water. Sometimes excess of hydroxyl ions, phosphate, and organic acids in water causes alkalinity. High alkalinity imparts bitter taste. The acceptable limit of alkalinity is 200 mg/l. Alkalinity of the samples was in range 240-444 mg/l. High alkalinity of 448 and 444 mg/l was observed at the inlet of wetlands (or outlet of the treatment plant). These values declined after the water passed through wetlands (in particular the algal pond) and also in the middle of Jakkur lake.
Total Hardness
Hardness is a measure of dissolved minerals that decides the utility of water for domestic purposes. Hardness is mainly due to the presence of carbonates and bicarbonates that is temporary hardness and due to sulphates and chlorides that is permanent hardness. It is caused by variety of dissolved polyvalent metallic ions predominantly calcium and magnesium cation or other cations like barium, iron, manganese, strontium and zinc. In the present study, the total hardness ranged between 198 to 256 mg/l. It was higher in the inlets. High values of hardness are probably due to the regular addition of sewage and detergents.
Calcium
Calcium (Ca) is one amongst the major macro nutrients which are needed for the growth, development and reproduction in case of both plants and animals. The presence of Ca in water is mainly due to its passage through deposits of limestone, dolomite, gypsum and other gypsiferous materials (APHA 1998) along with the Ca (from sewage). It contributes to the total hardness of the water. Ca concentration in all samples analyzed periodically ranged between 39 to 71mg/l. Ca concentration was high in the sewage water (treated and untreated) entering into the lake.
Magnesium
Magnesium (Mg) in one of the most essential macro nutrients that helps as a co-factor in the enzyme systems and in the central metal ions that constitutes the chlorophyll molecule essential for plant photosynthesis. According to WHO guidelines, the maximum admissible limit is 50 mg/l. In this study, the concentration of Magnesium ranged from 19 - 26.82 mg/l.
Nutrients (Nitrates and Phosphates)
Nutrients essentially comprise of various forms of N and P that readily mineralizes (inorganic mineral ions) to enable uptake by microbes and plants. Accumulation of nitrates and inorganic P induces changes in water quality that affects its integrity leading to higher net productivity. Nitrates in excess amounts together with phosphates accelerate aquatic plant growth in surface water causing rapid oxygen depletion or eutrophication in the water. Nitrates at high concentrations (10 mg/l or higher) in surface and groundwater used for human consumption are particularly toxic to young children affecting the oxygen carrying capacity of blood cells (RBC) causing cyanosis (methemoglobinemia). In the present study, nitrate values ranged from 0.2 to 0.38 mg/l and phosphate values ranged between 0.09 to 1.29 mg/l. The nitrate and phosphate values are higher at the wetlands inlets (Fig. 4a ) and significantly reduce after the passage through wetlands and algal pond as elucidated in Fig, 4 b.
BOD and COD
BOD and COD are important parameters that indicate the presence of organic content. Biochemical oxygen demand (BOD) is the amount of oxygen required by bacteria while stabilizing decomposable organic matter under aerobic conditions. It is required to assess the pollution of surface and ground water where contamination occurs due to disposal of domestic and industrial effluents. Chemical oxygen demand (COD) determines the oxygen required for chemical oxidation of most organic matter and oxidizable inorganic substances with the help of strong chemical oxidant. In conjunction with the BOD, COD test is helpful in indicating toxic conditions and the presence of biologically resistant organic substances (Sawyer and McCarty 1978). In this study, the BOD values ranged from 17-128 mg/l. There was reduction of sixty-six percent in BOD after the algal pond and twenty-three percent removal in the water which flows out of the lake. The COD values ranged from 16 to 161 mg/l. The COD reduced by forty-five percent in the algae pond and thirty-two percent in the lake as shown in Fig. 4b.
Integrated Wastewater Treatment System – Constructed Wetland with Algal Pond
The treatment of domestic sewage in natural systems such as constructed wetlands and lagoons is being practiced in developing nations. Significant advantages are its construction and operations are simple and economically viable, while supporting local people’s livelihood. Lagoon systems are associated with a high growth rate of phytoplankton that are beneficial and are caused by the influence of light and the continuous nutrient inflow. Algal growth contributes towards the treatment of wastewater by transforming dissolved nutrients into particle aggregates (biomass). Algal retention in the lagoon helps in the treatment, which has to be harvested at regular interval to ensure effective treatment. Treatment system consisting of constructed wetlands and algal pond help in the removal of nutrients (Mahapatra et al. 2011a, 2013a, b; Ramachandra et al. 2018a).
Emergent macrophytes (such as Typha) act as a filter in removing suspended matter and avoiding anaerobic conditions by the root zone oxidation and the dissolved nutrients would be taken up by the lagoon algae. This type of treatment helps in augmenting the existing treatment system in complete removal of nutrients and bacteria. The combination of wetlands (with macrophytes assemblages), algal lagoon and a sustained harvesting of algae and macrophytes would provide complete solution to wastewater treatment systems with minimal maintenance. An integrated wastewater treatment system functional at Jakkur Lake since 2009 is explained in Fig. 4a. The integration of sewage treatment plant with wetlands (consisting of reed bed and algal pond) has helped in sustained treatment of water for reuse. Integrated wetland system at Jakkur provides an opportunity to assess the efficacy of treatment apart from providing insights for replicating similar systems to address the impending water scarcity in the rapidly urbanising Bangalore.
Fig 4a: Integrated Wetlands System for managing water and waste water
Insights to the Efficacy of Treatment
The treatment plant (1.6 Ha) with an installed capacity of 10 MLD, comprises of an Upflow Anaerobic Sludge Blanket Reactor (UASB) with an extended aeration system for sewage treatment. The treatment effluent then gets into wetlands (settling basin) of spatial extent ~4.63 hecatres consisting of diverse macrophytes such as Typha sp., Cyperus sp., Ludwigia sp., Alternanthera sp., Water hyacinth sp., etc. in the shallow region (with an area of ~1.8 hectares) followed by deeper algal basin (covering an area of about 2.8 hecatre). This being the significant functional component with macrophytes and algae jointly helps in the nutrient removal and wastewater remediation. The water from the settling basin flow passes through three sluices of which only the middle one is functional (with moderate flow). This water flows into Jakkur lake that spans over 45 hectares. There were notably less occurrence of floating macrophytes, except near the outfalls (~0.5 Ha) due to blockage of the ouflow channels by solid wastes and debris. These macrophytes are being managed by local fishermen. Water in the Jakkur lake is clear with abundant phytoplankton diversity and acceptable densities, which indicates of a healthy trophic status.
The nutrient analysis shows (illustrated in Fig. 4.2), that treatment happens due to immergent macrophytes of the wetlands and algae, which removes ~45Percent COD, ~66 Percent BOD, ~33 Percent NO3-N and ~40 Percent PO43—P. Jakkur lake treats the water and acts as the final level of treatment which shown as stage two that removes ~ 32 Percent COD, ~23Percent BOD, ~ 0.3 Percent NO3-N and ~34 Percent PO43—P. The synergestic mechanism of sewage treatment plants followed by wetlands helps in the complete removal of nutrients to acceptable levels according to CPCB norms.
Jakkur STP (of 10 MLD capacity) treats only 6 MLD of sewage that is drawn from Yelahanka town. Yet, it is observed that sewer channel carrying voluminous wastewater with the treatment plant effluents into wetlands. The major nutrient removal and polishing is done by the constructed wetlands and the lake. This wetland comprise of emergent macrophytes as Typha angustata, etc and plays a key role in oxygenation of soil subsystems through root zone oxidation and entrapment of necessary nutrients that otherwise would cause an algal bloom in the lake. The algal species in this manmade wetland region (Fig. 5) primarily comprised of members of chlorophyceae followed by cyanophyceae, euglenophyceae and bacillariophyceae (Fig. 6). The relative abundances are provided in the pie-diagrams (Fig. 6). The detailed list of algal species, their presence and absence have been listed and provided in Table 4.
Similarly macrophytes play an important role in the effluent stabilisation. The distribution of the macrophytes in the wetland area as well as at the outfalls of the lake is provided in Figure 7. Typha angustata species were dominating (54%) in the wetland area followed by Alternanthera philoxeroides (28%). However even though the macrophyte population was scarce in the lake, but still amongst them Eicchornia crassipes (84%) were dominating (Fig. 8), which were only restricted to the outlet reaches due to fish nets, deployed for fishing in core area.
Land use (LU) dynamics in wetlands catchment
Land use changes in the wetland catchments are the direct and indirect consequence of human actions to secure essential resources. These changes encompass the greatest environmental concerns of human populations today, including loss of biodiversity, pollution of water and soil, and changes in the climate. Monitoring and mitigating the negative consequences of LU changes, while sustaining the production of essential wetlands resources has therefore become a major priority today.
Land use change analyses are done using Landsat MSS (1973), IRS P6 data (2013) and Google Earth (http://earth.google.com). The Landsat data is cost effective, with high spatial resolution and freely downloadable from public domains like GLCF (http://glcfapp.glcf.umd.edu:8080/esdi/index.jsp) and USGS (http://glovis.usgs.gov/ ). IRS P6 LISS-IV (Indian Remote Sensing Satellite, part of the Indian Space Programme) data was procured from the National Remote Sensing Centre, Hyderabad (http://www.nrsc.gov.in).
Remote sensing data obtained were geo-referenced, rectified and cropped corresponding to the study area. Geo-registration of remote sensing data (Landsat data) has been done using ground control points collected from the field using pre calibrated GPS (Global Positioning System) and also from known points (such as road intersections, etc.) collected from geo-referenced topographic maps published by the Survey of India. In the correction process, numerous GCP’s are located in terms of their two image coordinates; on the distorted image and in terms of their ground coordinates typically measured from a map or located in the field, in terms of UTM coordinates as well as latitude and longitude. The Landsat data of 1973 are with a spatial resolution of 57.5 m x 57.5 m (nominal resolution), while IRS P6 are of 5.8 m.
Land use analyses involved (i) generation of False Color Composite (FCC) of remote sensing data (bands–green, red and NIR). This composite image helps in locating heterogeneous patches in the landscape, (ii) selection of training polygons by covering 15% of the study area (polygons are uniformly distributed over the entire study area) (iii) loading these training polygons co-ordinates into pre-calibrated GPS, (vi) collection of the corresponding attribute data (land use types) for these polygons from the field. GPS helped in locating respective training polygons in the field, (iv) supplementing this information with Google Earth and (v) 60Percent of the training data has been used for classification, while the balance is used for validation or accuracy assessment. The land use analysis was done using supervised classification technique based on Gaussian maximum likelihood algorithm with training data (collected from field using GPS).
Classifier based on Gaussian Maximum Likelihood algorithm has been widely applied as an appropriate and efficient classifier to extract information from remote sensing data. This approach quantitatively evaluates both the variance and covariance of the category spectral response patterns when classifying an unknown pixel of remote sensing data, assuming the distribution of data points to be Gaussian. After evaluating the probability in each category, the pixel is assigned to the most likely class (highest probability value). GRASS GIS (Geographical Resources Analysis Support System, http://ces.iisc.ernet.in/grass) a free and open source software with the robust support for processing both vector and raster data has been used for analyzing RS data. Temporal remote sensing data have been classified through supervised classification techniques by using available multi-temporal “ground truth” information. Earlier time data were classified using the training polygon along with attribute details compiled from the historical published topographic maps, vegetation maps, revenue maps, land records available from local administrative authorities.
Temporal remote sensing data of Landsat (1973) and IRS (2013) were classified into four land use categories (Fig. 9): tree vegetation, built-up, water-bodies and others (agriculture, open area, etc.). The analyses show decline of tree vegetation by 50Percent from 44.06Percent (1973) to 22.38Percent (2013), with an increase in built-up from 1.19 (1973) to 6.56Percent (2013). Details of land use analyses are listed in Table 5.
Integrated Wetlands Ecosystem: Sustainable Model to Mitigate Water Shortages in Bangalore
Performance assessment of an integrated wetland ecosystem at Jakkur provides vital insights towards mitigating water crisis in Bangalore. Functional aspects of the integrated wetlands systems are:
• Sewage Treatment Plant (STP): The purpose of sewage treatment is to remove contaminants (Carbon and solids) from sewage to produce an environmentally safe water. The treatment based on physical, chemical, and biological processes include three stages – primary, secondary and tertiary. Primary treatment entails holding the sewage temporarily in a settling basin to separate solids and floatables. The settled and floating materials are filtered before discharging the remaining liquid for secondary treatment to remove dissolved and suspended biological matter. STP’s effluents were still nutrient rich requiring further treatment (for nutrient removal) and stabilization for further water utilities in the vicinity.
• Integration with wetlands [consisting of reed (typha etc.) beds and algal pond] would help in the complete removal of nutrients in the cost effective way. A nominal residence time (~5 days) would help in the removal of pathogen apart from nutrients. However, this requires regular maintenance of harvesting macrophytes and algae (from algal ponds). Harvested algae would have energy value, which could be used for biofuel production. The wetland systems helps in the removal of ~77 Percent COD, ~90Percent BOD, ~33Percent NO3-N and ~75Percent PO43-P (Fig.10).
Pilot scale experiment in the laboratory has revealed nutrient removal of algae are 86Percent, 90Percent, 89Percent, 70Percent and 76Percent for TOC, TN, Amm.-N, TP and OP respectively (Fig. 11) and lipid content varied from 18-28.5 Percent of dry algal biomass. Biomass productivity is of ~122 mg/l/d and lipid productivity of ~32 mg/l/d. Gas chromatography and mass spectrometry (GC-MS) analysis of the fatty acid methyl esters (FAME) showed a higher content of desirable fatty acids (biofuel properties) with major contributions from saturates such as palmitic acid [C16:0; ~40%], stearic acid [C18:0; ~34%] followed by unsaturates as oleic acid [C18:1(9); ~10%] and linoleic acid [C18:2(9,12); ~5%]. The decomposition of algal biomass and reactor residues with calorific exothermic heat content of 123.4 J/g provides the scope for further energy derivation (Mahapatra et al., 2014). Water that comes out of the wetlands is portable with minimal efforts for pathogen removal via solar disinfection.
Our earlier experiments have shown the vital role of wetlands in recharging the groundwater resources, evident from the decline of groundwater table to 200-300 m (with the removal of wetlands) from 30 to 50 m. Jakkur lake system is helping in recharging the groundwater sources. There need to be regulation on the exploitation of groundwater in Bangalore. Over exploitation of groundwater through borewells by commercial private agencies would harm the sustainability, depriving the local residents in the vicinity who are dependent on borewells in the absence of piped water supply from the government agency. Measures required to mitigate water crisis in burgeoning Bangalore are:
i. Rainwater harvesting at decentralized levels through wetlands (lakes) is the most efficient and cost effective mechanism to address the water crisis in the region than technically infeasible, ecologically unsound and economically unviable river diversion or inter linking of river schemes being proposed by vested interests in various parts of the country.
ii. Rejuvenation, restoration of existing lakes. This is necessary to decontaminate water bodies due to the unabated inflow of effluents and sewage.
iii. Removal of deposited silt would enhance the storage capacity as well as bioremediation capability of lakes.
iv. Integrated wetlands ecosystem (consisting of reed bed (typha, etc.) and algal pond) with lake helps in the treatment of water entering the lake through bioremediation. Replicating Jakkur wetland ecosystem would help in the treatment of water and reuse. This also has an added advantage of maintaining groundwater quality in the vicinity. Studies have shown that groundwater sources in the vicinity of sewage fed lakes are contaminated, evident from the nutrient enrichment, presence of coliform, etc.
v. Sustainable management of integrated wetlands ecosystem includes
vi. Letting only secondary treated sewage to wetlands.
vii. Maintaining at-least 33% vegetation cover in the lake catchment. This is necessary to ensure sufficient infiltration of rainwater to ensure water in the lake throughout the year.
viii. Ban on number of bore wells (or extraction of groundwater) in the lake catchment and command area.
ix. Restriction on overexploitation of groundwater in the lake catchment to ensure sustained water availability to the local residents.
x. Regular harvesting of macrophytes.
xi. Mechanism to harvest algae at regular interval and manufacture of biofuel and other beneficial biochemical products. These would enhance the employment opportunity in the region.
xii. Provision of appropriate infrastructure for washer men who depend on the lake for livelihood through washing clothes.
xiii. Restriction on the introduction of exotic species of fish by commercial vendors.
xiv. Permission to scientific fish culturing through strict regulations (on fish species introduction, type of nets, frequency of harvesting, restrictions during breeding season and locations).
Area Required for Constructed Wetlands
Taking advantage of remediation capability of aquatic plants (emergent macrophytes, free floating macrophytes) and algae, constructed wetlands have been designed and implemented successfully for efficient removal of nutrients (N, P, heavy metals, etc.). Different types of constructed wetlands (sub surface 0.6 m depth, surface: 0.4 m, could be either horizontal or vertical). Area required for constructed wetlands depends on the influent sewage quality and expected treatment (BOD removal, etc.) is given in equation 1 (Vymazal et al. 1998). Estimates show that to treat 1 MLD influent, area required is about 1.7 hectares. Figure 11 gives the proposed design of wetlands to treat 1 MLD.
Where,
A = area; Qd= ave flow (m3/day); Co and Ct = influent and effluent BOD (mg/L); KBOD = 0.10
For example to treat influent (raw sewage: BOD: 60-80) and anticipated effluent (with BOD 10), area required is about 1.7 to 2 hectares.
|
|
Citation : Ramachandra T V, Sincy V, Asulabha K S, Mahapatra D M, Sudarshan P B and Bharath H Aithal, 2018. Optimal Treatment of Domestic Wastewater through Constructed Wetlands, Journal of Biodiveristy, 9(1&2): 81-102
|